14 & Electrolytes
Learning Objectives
At the end of this unit, you should be able to:
- Define and describe the measurement of pH.
- Describe the electrolyte composition of the body.
- Describe the mechanisms for the control of electrolytes in the body.
Learning Objectives and Guiding Questions
At the end of this unit, you should be able to complete all the following tasks, including answering the guiding questions associated with each task.
- Describe the difference between compounds that are electrolytes and those that are non-electrolytes.
- Specify four functions of ions in the body.
- Compare and contrast the electrolyte composition of each of the following pairs of body fluid compartments:
- Intracellular fluid and blood plasma
- Intracellular fluid and interstitial fluid
- Blood plasma and interstitial fluid
- Of the three major fluid compartments in the human body (intracellular fluid, blood plasma, and interstitial fluid), which two are most similar to each other? Explain why this grouping is not unexpected.
- Define the following terms: electrolyte, acid, base, buffer.
- Define and clearly distinguish between an electrolyte, an acid, and a base.
- Explain the chemical nature of a buffer solution. Describe the function of such solutions in the human body and explain how they perform that function.
- Describe pH and the pH scale.
- Describe how to use the pH scale to determine hydrogen ion concentration, and how to use it to compare two solutions, by completing the following questions:
- Explain in words how to calculate the difference in hydrogen ion concentration between a solution with a pH of 4 and one with a pH of 9.
- State whether each solution described above is acidic, basic or neutral.
- For each of the following solutions, state (a) whether it is acidic or basic, AND (b) whether it is “more acidic” or “less acidic” than a solution with a pH of 7.3:
- A solution with a pH of 7.2
- A solution with a pH of 6.4
- A solution with a pH of 7.8
- Describe the sources of acids and bases in the body.
- Describe the mechanism(s) by which acids and/or bases arise in the human body as a result of each of the following:
- Introduction of regular foodstuffs into the digestive tract
- Ingestion of an antacid tablet
- Anaerobic conditions (lactic acid fermentation)
- Aerobic cellular respiration
- Amino acid catabolism
- Triglyceride catabolism
- Gluconeogenesis
- Describe three mechanisms by which the body regulates its pH.
- Describe the carbonic acid-bicarbonate buffer system and specify two other buffer systems.
- Define acidosis and specify two general causes of acidosis.
- Define alkalosis and specify two general causes of alkalosis.
- Compare and contrast (describe similarities and differences between):
- Acidosis and alkalosis
- Respiratory acidosis and respiratory alkalosis
- Metabolic acidosis and metabolic alkalosis
- Respiratory acidosis and metabolic acidosis
- Metabolic alkalosis and respiratory alkalosis
- Specify at least two causative disorders or circumstances that would cause each of the following and explain how they do so.
- Respiratory alkalosis
- Respiratory acidosis
- Metabolic alkalosis
- Metabolic acidosis
Body Fluids and Fluid Compartments
The chemical reactions of life take place in aqueous solutions. The dissolved substances in a solution are called solutes. In the human body, solutes vary in different parts of the body, but may include proteins—including those that transport lipids, carbohydrates, and very importantly, electrolytes.
In the body, water moves through semi-permeable membranes of cells and from one compartment of the body to another by osmosis. An appropriate balance of solutes inside and outside of cells must be maintained to ensure normal function.
Body Water Content: Human beings are mostly water, ranging from about 75 percent of body mass in infants to about 50–60 percent in adult men and women, to as low as 45 percent in old age.
Fluid Compartments: Body fluids can be discussed in terms of their specific fluid compartment, a location that is largely separate from another compartment by some form of a physical barrier. The intracellular fluid (ICF) compartment is the system that includes all fluid enclosed in cells by their plasma membranes. Extracellular fluid (ECF) surrounds all cells in the body. Extracellular fluid has two primary constituents: the fluid component of the blood (called plasma) and the interstitial fluid (IF) that surrounds all cells not in the blood (Figure 1).
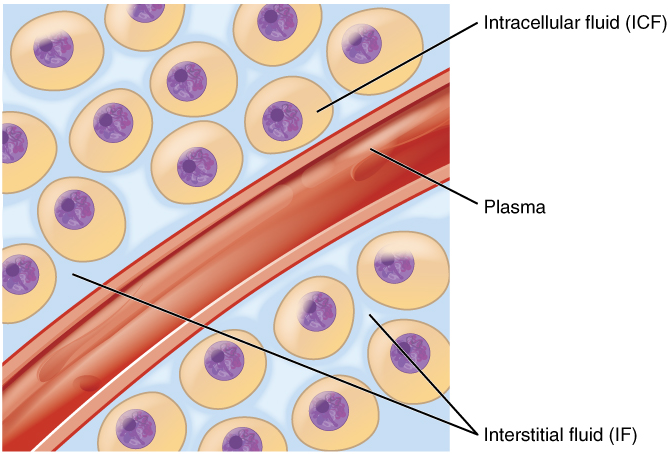
Intracellular Fluid: The intracellular fluid lies within cells and is the principal component of the cytosol. The intracellular fluid makes up about 60 percent of the total water in the human body (Figure 2). The amount of water in living cells is closely regulated. If the amount of water inside a cell falls to a value that is too low, the cytosol becomes too concentrated with solutes to carry on normal cellular activities; if too much water enters a cell, the cell may burst and be destroyed.
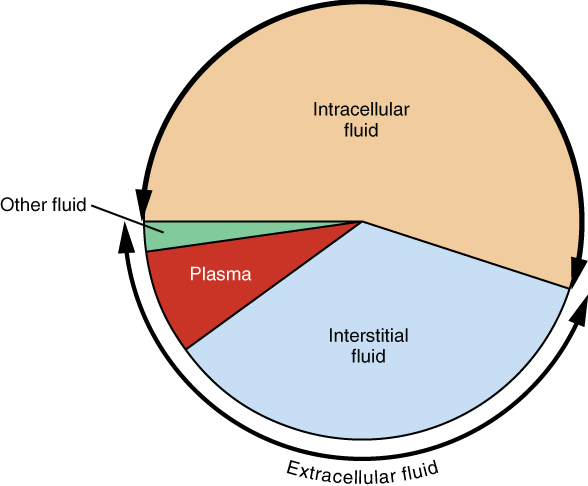
Extracellular Fluid: The extracellular fluid accounts for the other one-third of the body’s water content. Approximately 20 percent of the extracellular fluid is found in plasma. Plasma travels through the body in blood vessels and transports a range of materials, including blood cells, proteins (including clotting factors and antibodies), electrolytes, nutrients, gases, and wastes. Gases, nutrients, and waste materials travel between capillaries and cells through the interstitial fluid. Cells are separated from the interstitial fluid by a selectively permeable cell membrane that helps regulate the passage of materials between the interstitial fluid and the interior of the cell.
The body has other water based extracellular fluid. These include the cerebrospinal fluid that bathes the brain and spinal cord, lymph, the synovial fluid in joints, the pleural fluid in the pleural cavities, the pericardial fluid in the cardiac sac, the peritoneal fluid in the peritoneal cavity, and the aqueous humor of the eye. Because these fluids are outside of cells, these fluids are also considered components of the extracellular fluid compartment.
Composition of Body Fluids: The compositions of the two components of the extracellular fluid—plasma and interstitial fluid—are more similar to each other than either is to the intracellular fluid (Figure 3). Blood plasma has high concentrations of sodium, chloride, bicarbonate, and protein. The interstitial fluid has high concentrations of sodium, chloride, and bicarbonate, but a relatively lower concentration of protein. In contrast, the intracellular fluid has elevated amounts of potassium, phosphate, magnesium, and protein. Overall, the intracellular fluid contains high concentrations of potassium and phosphate (HPO42−), whereas both plasma and the extracellular fluid contain high concentrations of sodium and chloride.
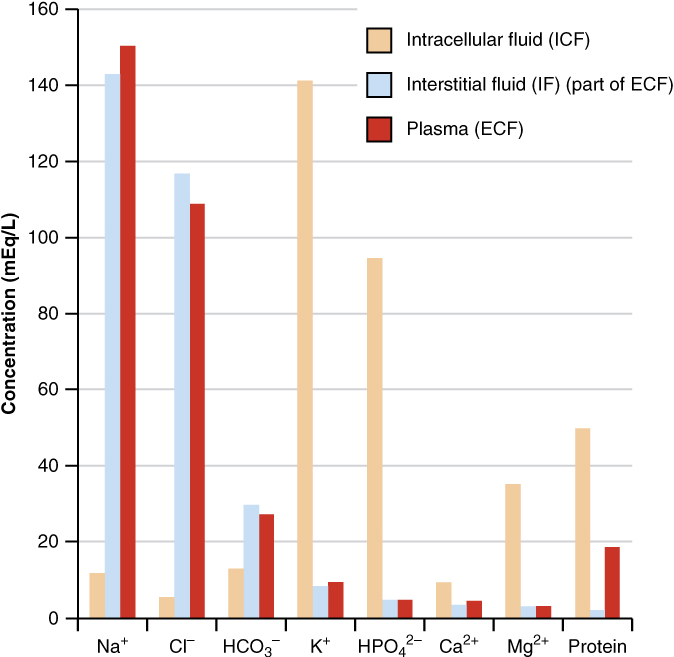
Body fluids are neutral in charge. Thus, cations, or positively charged ions, and anions, or negatively charged ions, are balanced in fluids. As seen in the previous graph, sodium (Na+) ions and chloride (Cl–) ions are concentrated in the extracellular fluid of the body, whereas potassium (K+) ions are concentrated inside cells. Although sodium and potassium can “leak” through “pores” into and out of cells, respectively, the high levels of potassium and low levels of sodium in the intracellular fluid are maintained by sodium-potassium pumps in the cell membranes. These pumps use the energy supplied by ATP to pump sodium out of the cell and potassium into the cell (Figure 4).
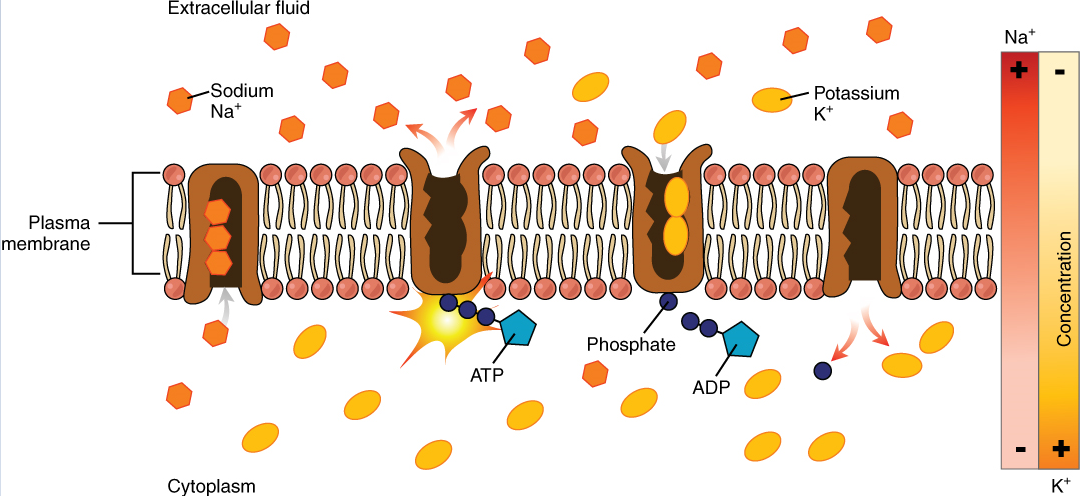
Roles of Electrolytes: The body contains a large variety of ions, or electrolytes, which perform a variety of functions. Some ions assist in the transmission of electrical impulses along cell membranes in neurons and muscles. Other ions help to stabilize protein structures in enzymes. Still others aid in releasing hormones from endocrine glands. All of the ions in plasma contribute to the osmotic balance that controls the movement of water between cells and their environment.
Electrolytes in living systems include sodium, potassium, chloride, bicarbonate, calcium, phosphate, magnesium, copper, zinc, iron, manganese, molybdenum, copper, and chromium. In terms of body functioning, six electrolytes are most important: sodium, potassium, chloride, bicarbonate, calcium, and phosphate.
These six ions aid in nerve excitability, endocrine secretion, membrane permeability, buffering body fluids, and controlling the movement of fluids between compartments. These ions enter the body through the digestive tract. More than 90 percent of the calcium and phosphate that enters the body is incorporated into bones and teeth, with bone serving as a mineral reserve for these ions. In the event that calcium and phosphate are needed for other functions, bone tissue can be broken down to supply the blood and other tissues with these minerals. Phosphate is a normal constituent of nucleic acids; hence, blood levels of phosphate will increase whenever nucleic acids are broken down.
Excretion of ions occurs mainly through the kidneys, with lesser amounts lost in sweat and in feces. Excessive sweating may cause a significant loss, especially of sodium and chloride. Severe vomiting or diarrhea will cause a loss of chloride and bicarbonate ions. Adjustments in respiratory and renal functions allow the body to regulate the levels of these ions in the extracellular fluid.
Acid-Base Balance
Acids and bases, like salts, dissociate in water into electrolytes. Acids and bases can very much change the properties of the solutions in which they are dissolved.
Acids: An acid is a substance that releases hydrogen ions (H+) in solution (Figure 5a). Because an atom of hydrogen has just one proton and one electron, a positively charged hydrogen ion is simply a proton. This solitary proton is highly likely to participate in chemical reactions. Strong acids are compounds that release all of their H+ in solution; that is, they ionize completely. Hydrochloric acid (HCl), which is released from cells in the lining of the stomach, is a strong acid because it releases all of its H+ in the stomach’s watery environment. This strong acid aids in digestion and kills ingested microbes. Weak acids do not ionize completely; that is, some of their Hydrogen ions remain bonded within a compound in solution. An example of a weak acid is vinegar, or acetic acid; it is called acetate after it gives up a proton. Other common examples used in cellular metabolism include pyruvic acid, citric acid, and oxaloacetic acid which in water may release a proton to become pyruvate, citrate, and oxaloacetate respectively.
Bases: A base is a substance that releases hydroxyl ions (OH–) in solution, or one that accepts H+ already present in solution (Figure 5b). The hydroxyl ions (also known as hydroxide ions) or other basic substances combine with H+ present to form a water molecule, thereby removing H+ and reducing the solution’s acidity. Strong bases release most or all of their hydroxyl ions; weak bases release only some hydroxyl ions or absorb only a few H+
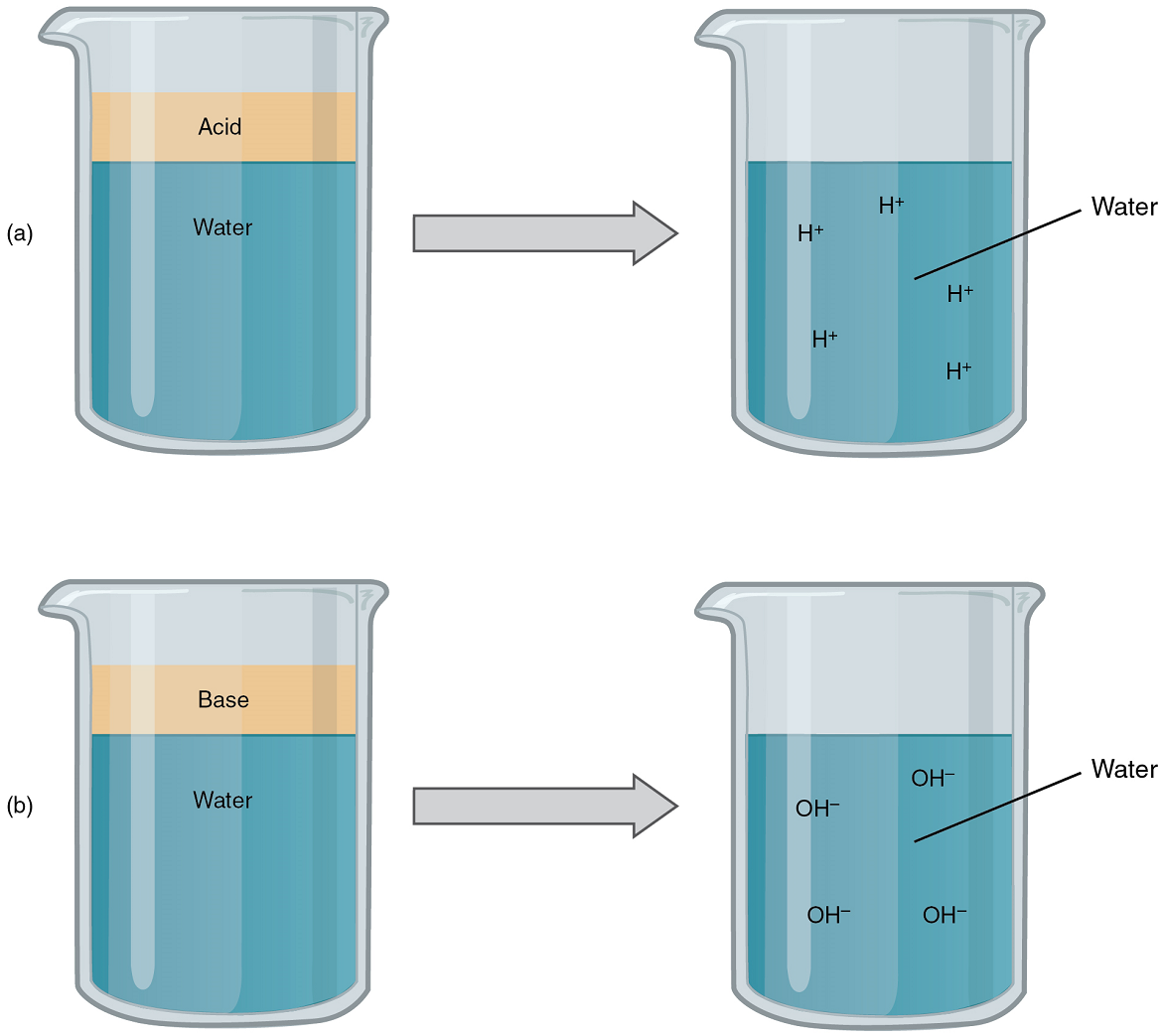
The Concept of pH: The relative acidity or alkalinity of a solution can be indicated by its pH. pH literally means the “potential of hydrogen”. It is a measure of the amount of hydrogen ions present per litre of a solution, expressed in grams. In technical terms, pH is the logarithm of the reciprocal of the hydrogen ion concentration of a solution.
As an example, a pH 4 solution has an H+ concentration that is ten times greater than that of a pH 5 solution. That is, a solution with a pH of 4 is ten times more acidic than a solution with a pH of 5. The concept of pH will begin to make more sense when you study the pH scale (Figure 6). The scale consists of a series of increments ranging from 0 to 14. A solution with a pH of 7 is considered neutral—neither acidic nor basic. Pure water has a pH of 7. The lower the number below 7, the more acidic the solution, or the greater the concentration of H+. The concentration of hydrogen ions at each pH value is 10 times different than the next pH. The higher the number above 7, the more basic (alkaline) the solution, or the lower the concentration of H+.
Buffers: The pH of human blood normally ranges from 7.35 to 7.45. At this slightly basic pH, blood can reduce the acidity resulting from the carbon dioxide (CO2) constantly being released into the bloodstream by the trillions of cells in the body. Homeostatic mechanisms (along with exhaling CO2 while breathing) normally keep the pH of blood within this narrow range. This is critical, because fluctuations—either too acidic or too alkaline—can lead to life-threatening disorders.
All cells of the body depend on homeostatic regulation of acid–base balance at a very narrow range of pH between 7.35 to 7.45. The body therefore has several mechanisms for this regulation, involving breathing, the excretion of chemicals in urine, and the internal release of chemicals collectively called buffers into body fluids. A buffer is a solution of a weak acid and its conjugate base. A buffer can resist sudden changes in the acidity and alkalinity of the body fluids. For example, if there is even a slight decrease below 7.35 in the pH of a bodily fluid, the buffer in the fluid—in this case, acting as a weak base—will bind the excess hydrogen ions.
In contrast, if pH rises above 7.45, the buffer will act as a weak acid and contribute hydrogen ions.
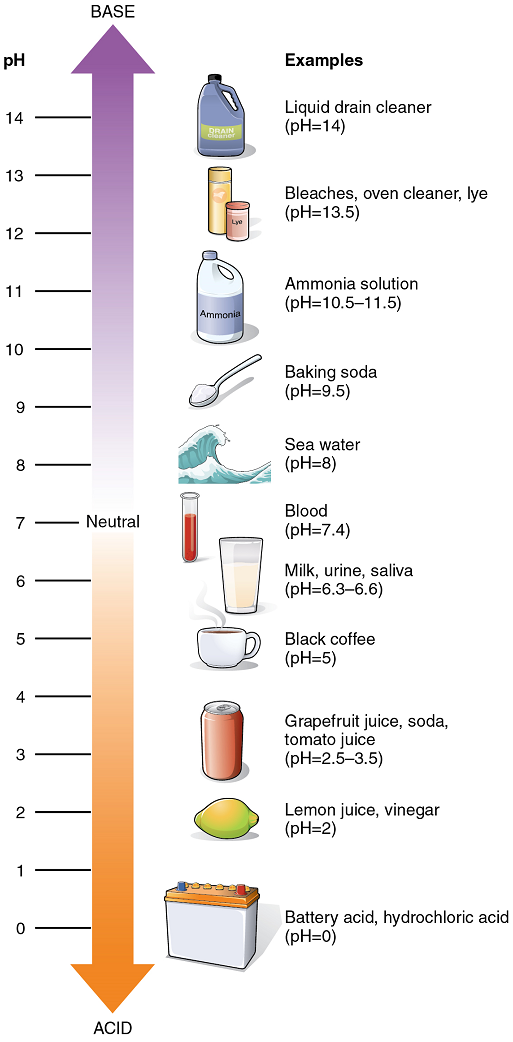
Acid-Base Balance: Proper physiological functioning depends on a very tight balance between the concentrations of acids and bases in the blood. Acid-base balance is measured using the pH scale (Figure 6). A variety of buffering systems permits blood and other bodily fluids to maintain a narrow pH range, even in the face of perturbations. A buffer is a chemical system that minimizes change in hydrogen ion concentration.
Buffer Systems in the Body: The buffer systems in the human body are extremely efficient, and different systems work at different rates. It takes only seconds for the chemical buffers in the blood to resist changes in the pH. The respiratory tract can adjust the blood pH upward in minutes by exhaling CO2 from the body. The renal system can also adjust blood pH through the excretion of hydrogen ions (H+) and the conservation of bicarbonate, but this process takes hours to days to have an effect.
The buffer systems functioning in blood plasma include plasma proteins, phosphate, and bicarbonate and carbonic acid buffers. The kidneys help control acid-base balance by excreting hydrogen ions and generating bicarbonate that helps maintain blood plasma pH within a normal range.
Protein Buffers in Blood Plasma and Cells: Protein buffer systems work predominantly inside cells. Nearly all proteins can function as buffers. Proteins are made up of amino acids, which contain positively charged amino groups and negatively charged carboxyl groups. The charged regions of free amino acids can bind hydrogen and hydroxyl ions, and thus function as buffers. Buffering by proteins accounts for two-thirds of the buffering power of the blood and most of the buffering within cells.
Phosphate Buffer: Phosphates are found in the blood in two forms: sodium dihydrogen phosphate (NaH2PO4), which is a weak acid, and sodium monohydrogen phosphate (Na2HPO4), which is a weak base. When Na2HPO4 comes into contact with a strong acid, such as HCl, the base reacts with the hydrogen ion released by the HCl to form the weak acid NaH2PO4 and sodium chloride (NaCl). When NaH2PO4 (the weak acid) comes into contact with a strong base, such as sodium hydroxide (NaOH), the weak acid releases H+ ions which bind to the OH– ions released by the base to produce water.
HCl + Na2HPO4→NaH2PO4 + NaCl
(strong acid) + (weak base) → (weak acid) + (a salt)
NaOH + NaH2PO4→Na2HPO4 + H2O
(strong base) + (weak acid) → (weak base) + (water)
Bicarbonate-Carbonic Acid Buffer: The bicarbonate-carbonic acid buffer works in a fashion similar to phosphate buffers. When sodium bicarbonate (NaHCO3), comes into contact with a strong acid, such as HCl, carbonic acid (H2CO3), which is a weak acid, and NaCl are formed. When carbonic acid comes into contact with a strong base, such as NaOH, bicarbonate and water are formed.
NaHCO3 + HCl → H2CO3 + NaCl
(sodium bicarbonate) + (strong acid) → (weak acid) + (a salt)
H2CO3 + NaOH→ NaHCO3 + H2O
(weak acid) + (strong base) → (sodium bicarbonate) + (water)
As with the phosphate buffer, a weak acid or weak base captures the free ions, and a significant change in pH is prevented. Bicarbonate ions and carbonic acid are present in the blood in a 20:1 ratio if the blood pH is within the normal range. With 20 times more bicarbonate than carbonic acid, this capture system is most efficient at buffering changes that would make the blood more acidic. This is useful because most of the body’s metabolic wastes, such as lactic acid and ketone bodies, are acids. Carbonic acid levels in the blood are controlled by the expiration of CO2 through the lungs. The level of bicarbonate in the blood is controlled through the renal system, where bicarbonate ions in the renal filtrate are conserved and passed back into the blood. However, the bicarbonate buffer is the primary buffering system of the interstitial fluid surrounding the cells in tissues throughout the body.
Respiratory Regulation of Acid-Base Balance: The respiratory system contributes to the balance of acids and bases in the body by regulating the blood levels of carbonic acid (Figure 7). CO2 in the blood readily reacts with water to form carbonic acid, and the levels of CO2 and carbonic acid in the blood are in equilibrium. When the CO2 level in the blood rises (as it does when you hold your breath), the excess CO2 reacts with water to form additional carbonic acid, lowering blood pH. Increasing the rate and/or depth of respiration (which you might feel the “urge” to do after holding your breath) allows you to exhale more CO2. The loss of CO2 from the body reduces blood levels of carbonic acid and thereby adjusts the pH upward, toward normal levels. This process also works in the opposite direction, excessive deep and rapid breathing (as in hyperventilation) rids the blood of CO2 and reduces the level of carbonic acid, making the blood too alkaline.
Renal Regulation of Acid-Base Balance: The renal regulation of the body’s acid-base balance addresses the metabolic component of the buffering system. Whereas the respiratory system (together with breathing centers in the brain) controls the blood levels of carbonic acid by controlling the exhalation of CO2, the renal system controls the blood levels of bicarbonate.
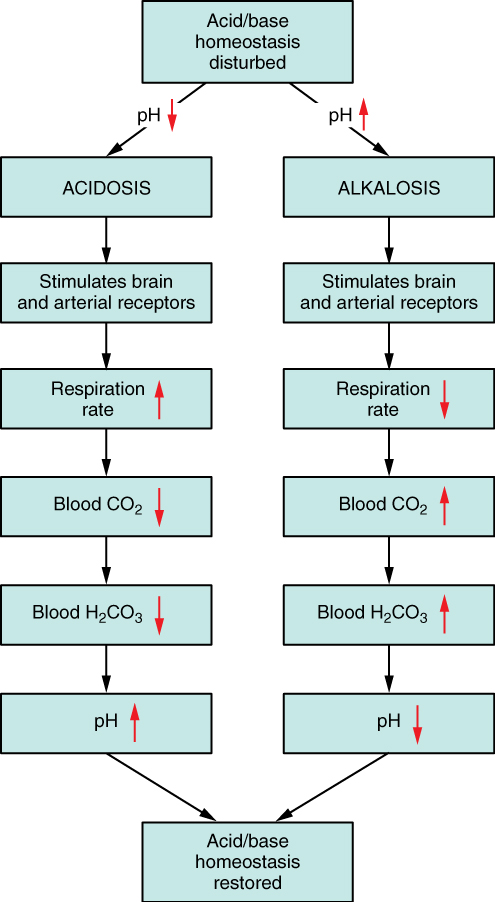
A decrease of blood bicarbonate can result by certain diuretics or from excessive bicarbonate loss due to diarrhea. Low bicarbonate blood levels can also occur as a result of elevated levels of ketone bodies (common in unmanaged diabetes mellitus), which bind bicarbonate hence, lowering their concentration in the blood.
Disorders of Acid-Base Balance: Normal arterial blood pH is restricted to a very narrow range of 7.35 to 7.45. A person who has a blood pH below 7.35 is considered to be in acidosis, and a continuous blood pH below 7.0 can be fatal. Acidosis has several symptoms, including headache and confusion, and the individual can become lethargic and easily fatigued (Figure 8). A person who has a blood pH above 7.45 is considered to be in alkalosis, and a pH above 7.8 is fatal. Some symptoms of alkalosis include cognitive impairment (which can progress to unconsciousness), tingling or numbness in the extremities, muscle twitching and spasm, and nausea and vomiting. Both acidosis and alkalosis can be caused by either metabolic or respiratory disorders.
As discussed earlier in this chapter, the concentration of carbonic acid in the blood is dependent on the level of CO2 in the body and the amount of CO2 gas exhaled through the lungs. Thus, the respiratory contribution to acid-base balance is usually discussed in terms of CO2 (rather than of carbonic acid). Remember that a molecule of carbonic acid is lost for every molecule of CO2 exhaled, and a molecule of carbonic acid is formed for every molecule of CO2 retained.
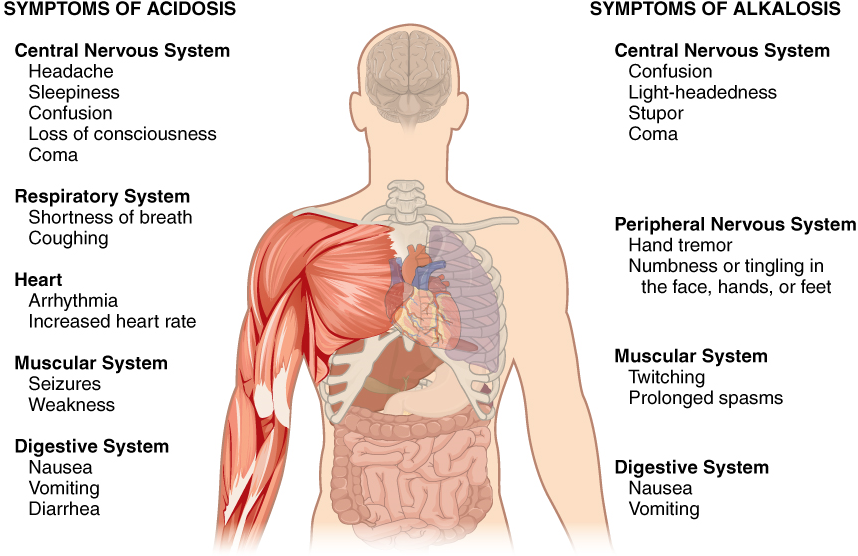
Metabolic Acidosis: Primary Bicarbonate Deficiency: Metabolic acidosis occurs when the blood is too acidic (pH below 7.35) due to too little bicarbonate in the body fluids. At the normal pH of 7.40, the ratio of bicarbonate to carbonic acid buffer is 20:1. If a person’s blood pH drops below 7.35, then he or she is in metabolic acidosis. The most common cause of metabolic acidosis is the presence of organic acids or excessive ketone bodies in the blood. Table 1 lists some other causes of metabolic acidosis.
The first three of the eight causes of metabolic acidosis listed are medical (or unusual physiological) conditions. Strenuous exercise can cause temporary metabolic acidosis due to the production of lactic acid. The last five causes result from the ingestion of specific substances. The active form of aspirin is its metabolite, acetylsalicylic acid. An overdose of aspirin causes acidosis due to the acidity of this drug. Metabolic acidosis can also result from uremia, which is the retention of urea and uric acid. Metabolic acidosis can also arise from diabetic ketoacidosis, wherein an excess of ketones is present in the blood. Other causes of metabolic acidosis are a decrease in the excretion of hydrogen ions, which inhibits the conservation of bicarbonate ions, and excessive loss of bicarbonate ions through the gastrointestinal tract due to diarrhea.
Cause | Metabolite affected |
---|---|
Diarrhea | Bicarbonate |
Uremia | Phosphoric, sulfuric, lactic acids |
Diabetic ketoacidosis | Ketones |
Strenuous exercise | Lactic acid |
Methanol ingestion | Formic acid (metabolite of methanol) |
Paraldehyde ingestion | β-Hydroxybutyric acid (metabolite of paraldehyde) |
Isopropanol ingestion | Propionic acid (metabolite of isopropanol) |
Ethylene glycol ingestion | Glycolic acid, oxalic & formic acids (metabolites of ethylene glycol) |
Salicylate/aspirin ingestion | Sulfasalicylic acid (metabolite of salicylate) |
Metabolic Alkalosis: Primary Bicarbonate Excess: Metabolic alkalosis is the opposite of metabolic acidosis. It occurs when the blood is too alkaline (pH above 7.45) due to an excess of bicarbonate in the body fluids.
A transient excess of bicarbonate in the blood can follow ingestion of excessive amounts of bicarbonate, citrate, or antacids for conditions such as stomach acid reflux—known as heartburn. Other causes of metabolic alkalosis include the loss of hydrochloric acid from the stomach through vomiting, potassium depletion due to the use of diuretics for hypertension, and the excessive use of laxatives.
Respiratory Acidosis: Primary Carbonic Acid/CO2 Excess: Respiratory acidosis occurs when the blood is overly acidic due to an excess of carbonic acid, resulting from too much CO2 in the blood. Respiratory acidosis can result from anything that interferes with respiration, such as pneumonia, emphysema, or congestive heart failure.
Respiratory Alkalosis: Primary Carbonic Acid/CO2 Deficiency: Respiratory alkalosis occurs when the blood is overly alkaline due to a deficiency in carbonic acid and CO2 levels in the blood. This condition usually occurs when too much CO2 is exhaled from the lungs, as occurs in hyperventilation, which is breathing that is deeper or more frequent than normal. An elevated respiratory rate leading to hyperventilation can be due to extreme emotional upset or fear, fever, infections, hypoxia, or abnormally high levels of catecholamines, such as epinephrine and norepinephrine.
Compensation Mechanisms: Various compensatory mechanisms exist to maintain blood pH within a narrow range, including buffers, respiration, and renal mechanisms. Although compensatory mechanisms usually work very well, when one of these mechanisms is not working properly (like kidney failure or respiratory disease), they have their limits. If the pH and bicarbonate to carbonic acid ratio are changed too drastically, the body may not be able to compensate. Moreover, extreme changes in pH can denature proteins. Extensive damage to proteins in this way can result in disruption of normal metabolic processes, serious tissue damage, and ultimately death.
Respiratory Compensation: Respiratory compensation for metabolic acidosis increases the respiratory rate to drive off CO2 and readjust the bicarbonate to carbonic acid ratio to the 20:1 level. This adjustment can occur within minutes. Respiratory compensation for metabolic alkalosis is not as adept as its compensation for acidosis. The normal response of the respiratory system to elevated pH is to increase the amount of CO2 in the blood by decreasing the respiratory rate to conserve CO2. The respiratory route is less efficient at compensating for metabolic alkalosis than for acidosis.
Metabolic Compensation: Metabolic and renal compensation for respiratory diseases that can create acidosis revolves around the conservation of bicarbonate ions. In cases of respiratory acidosis, the kidney increases the conservation of bicarbonate and secretion of H+. These processes increase the concentration of bicarbonate in the blood, reestablishing the proper relative concentrations of bicarbonate and carbonic acid. In cases of respiratory alkalosis, the kidneys decrease the production of bicarbonate and conserve H+ ions.
Attribution Note: Chapter remixed from Douglas College Human Anatomy & Physiology I by the Douglas College Biology Department.